Greenland Ice Cap
Greenland has had major glaciers and ice caps for at least 18 million years, but a single ice sheet first covered most of the island some 2.6 million years ago. Since then, it has both grown and contracted significantly. The oldest known ice on Greenland is about 1 million years old. Due to anthropogenic greenhouse gas emissions, the ice sheet is now the warmest it has been in the past 1000 years, and is losing ice at the fastest rate in at least the past 12,000 years.
Every summer, parts of the surface melt and ice cliffs calve into the sea. Normally the ice sheet would be replenished by winter snowfall, but due to global warming the ice sheet is melting two to five times faster than before 1850, and snowfall has not kept up since 1996. If the Paris Agreement goal of staying below 2 °C (3.6 °F) is achieved, melting of Greenland ice alone would still add around 6 cm (2+1⁄2 in) to global sea level rise by the end of the century. If there are no reductions in emissions, melting would add around 13 cm (5 in) by 2100, with a worst-case of about 33 cm (13 in). For comparison, melting has so far contributed 1.4 cm (1⁄2 in) since 1972, while sea level rise from all sources was 15–25 cm (6–10 in) between 1901 and 2018.
If all 2,900,000 cubic kilometres (696,000 cu mi) of the ice sheet were to melt, it would increase global sea levels by ~7.4 m (24 ft). Global warming between 1.7 °C (3.1 °F) and 2.3 °C (4.1 °F) would likely make this melting inevitable. However, 1.5 °C (2.7 °F) would still cause ice loss equivalent to 1.4 m (4+1⁄2 ft) of sea level rise, and more ice will be lost if the temperatures exceed that level before declining. If global temperatures continue to rise, the ice sheet will likely disappear within 10,000 years. At very high warming, its future lifetime goes down to around 1,000 years.
Description

Ice sheets form through a process of glaciation, when the local climate is sufficiently cold that snow is able to accumulate from year to year. As the annual snow layers pile up, their weight gradually compresses the deeper levels of snow to firn and then to solid glacier ice over hundreds of years. Once the ice sheet formed in Greenland, its size remained similar to its current state. However, there have been 11 periods in Greenland's history when the ice sheet extended up to 120 km (75 mi) beyond its current boundaries; with the last one around 1 million years ago.

The weight of the ice causes it to slowly "flow", unless it is stopped by a sufficiently large obstacle, such as a mountain. Greenland has many mountains near its coastline, which normally prevent the ice sheet from flowing further into the Arctic Ocean. The 11 previous episodes of glaciation are notable because the ice sheet grew large enough to flow over those mountains. Nowadays, the northwest and southeast margins of the ice sheet are the main areas where there are sufficient gaps in the mountains to enable the ice sheet to flow out to the ocean through outlet glaciers. These glaciers regularly shed ice in what is known as ice calving. Sediment released from calved and melting ice sinks accumulates on the seafloor, and sediment cores from places such as the Fram Strait provide long records of glaciation at Greenland.
Geological history

While there is evidence of large glaciers in Greenland for most of the past 18 million years, these ice bodies were probably similar to various smaller modern examples, such as Maniitsoq and Flade Isblink, which cover 76,000 and 100,000 square kilometres (29,000 and 39,000 sq mi) around the periphery. Conditions in Greenland were not initially suitable for a single coherent ice sheet to develop, but this began to change around 10 million years ago, during the middle Miocene, when the two passive continental margins which now form the uplands of West and East Greenland experienced uplift, and ultimately formed the upper planation surface at a height of 2000 to 3000 meter above sea level.
Later uplift, during the Pliocene, formed a lower planation surface at 500 to 1000 meters above sea level. A third stage of uplift created multiple valleys and fjords below the planation surfaces. This uplift intensified glaciation due to increased orographic precipitation and cooler surface temperatures, allowing ice to accumulate and persist. As recently as 3 million years ago, during the Pliocene warm period, Greenland's ice was limited to the highest peaks in the east and the south. Ice cover gradually expanded since then, until the atmospheric CO2 levels dropped to between 280 and 320 ppm 2.7–2.6 million years ago, by which time temperatures had dropped sufficiently for the disparate ice caps to connect and cover most of the island.
Ice cores and sediment samples

The base of the ice sheet may be warm enough due to geothermal activity to have liquid water beneath it. This liquid water, under pressure from the weight of ice above it, may cause erosion, eventually leaving nothing but bedrock below the ice sheet. However, there are parts of the Greenland ice sheet, near the summit, where the ice sheet slides over a basal layer of ice which had frozen solid to the ground, preserving ancient soil, which can then be recovered by drilling. The oldest such soil was continuously covered by ice for around 2.7 million years, while another, 3 kilometres (1.9 mi) deep ice core from the summit has revealed ice that is around ~1,000,000 years old.
Sediment samples from the Labrador Sea provide evidence that nearly all of the south Greenland ice had melted around 400,000 years ago, during Marine Isotope Stage 11. Other ice core samples from Camp Century in northwestern Greenland, show that the ice there melted at least once during the past 1.4 million years, during the Pleistocene, and did not return for at least 280,000 years. These findings suggest that less than 10% of the current ice sheet volume was left during those geologically recent periods, when the temperatures were less than 2.5 °C (4.5 °F) warmer than preindustrial conditions. This contradicts how climate models typically simulate the continuous presence of solid ice under those conditions. Analysis of the ~100,000-year records obtained from 3 km (1.9 mi) long ice cores drilled between 1989 and 1993 into the summit of Greenland's ice sheet, had provided evidence for geologically rapid changes in climate, and informed research on tipping points such as in the Atlantic meridional overturning circulation (AMOC).

Ice cores provide valuable information about the past states of the ice sheet, and other kinds of paleoclimate data. Subtle differences in the oxygen isotope composition of the water molecules in ice cores can reveal important information about the water cycle at the time, while air bubbles frozen within the ice core provide a snapshot of the gas and particulate composition of the atmosphere through time. When properly analyzed, ice cores provide a wealth of proxies suitable for reconstructing the past temperature record, precipitation patterns, volcanic eruptions, solar variation, ocean primary production, and even changes in soil vegetation cover and the associated wildfire frequency. The ice cores from Greenland also record human impact, such as lead production during the time of Ancient Greece and the Roman Empire.
Recent melting

From the 1960s to the 1980s an area in the North Atlantic which included southern Greenland was one of the few locations in the world which showed cooling rather than warming. This location was relatively warmer in the 1930s and 1940s than in the decades immediately before or after. More complete data sets have established trends of warming and ice loss starting from 1900(well after the start of the Industrial Revolution and its impact on global carbon dioxide levels) and a trend of strong warming starting around 1979, in line with concurrent observed Arctic sea ice decline. In 1995– 1999, central Greenland was already 2 °C (3.6 °F) warmer than it was in the 1950s. Between 1991 and 2004, average winter temperature at one location, Swiss Camp, rose almost 6 °C (11 °F).
Consistent with this warming, the 1970s were the last decade when the Greenland ice sheet grew, gaining about 47 gigatonnes per year. From 1980–1990 there was an average annual mass loss of ~51 Gt/y. The period 1990–2000 showed an average annual loss of 41 Gt/y, with 1996 being the last year the Greenland ice sheet saw net mass gain. As of 2022, the Greenland ice sheet had been losing ice for 26 years in a row, and temperatures there had been the highest in the entire past last millennium – about 1.5 °C (2.7 °F) warmer than the 20th century average.
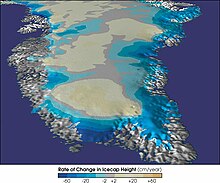
Several factors determine the net rate of ice sheet growth or decline. These are:
- Accumulation and melting rates of snow in and around the centre
- Melting of ice along the sheet's margins
- Ice calving into the sea from outlet glaciers also along the sheet's edges
When the IPCC Third Assessment Report was published in 2001, the analysis of observations to date had shown that the ice accumulation of 520 ± 26 gigatonnes per year was offset by runoff and bottom melting equivalent to ice losses of 297±32 Gt/yr and 32±3 Gt/yr, and iceberg production of 235±33 Gt/yr, with a net loss of −44 ± 53 gigatonnes per year.
Annual ice losses from the Greenland ice sheet accelerated in the 2000s, reaching ~187 Gt/yr in 2000–2010, and an average mass loss during 2010–2018 of 286 Gt per year. Half of the ice sheet's observed net loss (3,902 gigatons (Gt) of ice between 1992 and 2018, or approximately 0.13% of its total mass) happened during those 8 years. When converted to sea level rise equivalent, the Greenland ice sheet contributed about 13.7 mm since 1972.
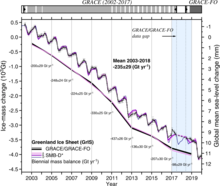
Between 2012 and 2017, it contributed 0.68 mm per year, compared to 0.07 mm per year between 1992 and 1997. Greenland's net contribution for the 2012–2016 period was equivalent to 37% of sea level rise from land ice sources (excluding thermal expansion). These melt rates are comparable to the largest experienced by the ice sheet over the past 12,000 years.
Currently, the Greenland ice sheet loses more mass every year than the Antarctic ice sheet, because of its position in the Arctic, where it is subject to intense regional amplification of warming. Ice losses from the West Antarctic Ice Sheet have been accelerating due to its vulnerable Thwaites and Pine Island Glaciers, and the Antarctic contribution to sea level rise is expected to overtake that of Greenland later this century.
Observed glacier retreat

Retreat of outlet glaciers as they shed ice into the Arctic is a large factor in the decline of Greenland's ice sheet. Estimates suggest that losses from glaciers explain between 49% and 66.8% of observed ice loss since the 1980s. Net loss of ice was already observed across 70% of the ice sheet margins by the 1990s, with thinning detected as the glaciers started to lose height. Between 1998 and 2006, thinning occurred four times faster for coastal glaciers compared to the early 1990s, falling at rates between 1 m (3+1⁄2 ft) and 10 m (33 ft) per year, while the landlocked glaciers experienced almost no such acceleration.
One of the most dramatic examples of thinning was in the southeast, at Kangerlussuaq Glacier. It is over 20 mi (32 km) long, 4.5 mi (7 km) wide and around 1 km (1⁄2 mi) thick, which makes it the third largest glacier in Greenland. Between 1993 and 1998, parts of the glacier within 5 km (3 mi) of the coast lost 50 m (164 ft) in height. Its observed ice flow speed went from 3.1–3.7 mi (5–6 km) per year in 1988–1995 to 8.7 mi (14 km) per year in 2005, which was then the fastest known flow of any glacier. The retreat of Kangerlussuaq slowed down by 2008, and showed some recovery until 2016–2018, when more rapid ice loss occurred.
Greenland's other major outlet glaciers have also experienced rapid change in recent decades. Its single largest outlet glacier is Jakobshavn Isbræ (Greenlandic: Sermeq Kujalleq) in west Greenland, which has been observed by glaciologists for many decades. It historically sheds ice from 6.5% of the ice sheet (compared to 4% for Kangerlussuaq), at speeds of ~20 metres (66 ft) per day. While it lost enough ice to retreat around 30 km (19 mi) between 1850 and 1964, its mass gain increased sufficiently to keep it in balance for the next 35 years, only to switch to rapid mass loss after 1997. By 2003, the average annual ice flow speed had almost doubled since 1997, as the ice tongue in front of the glacier disintegrated, and the glacier shed 94 square kilometres (36 sq mi) of ice between 2001 and 2005. The ice flow reached 45 metres (148 ft) per day in 2012, but slowed down substantially afterwards, and showed mass gain between 2016 and 2019.
Northern Greenland's Petermann Glacier is smaller in absolute terms, but experienced some of the most rapid degradation in recent decades. It lost 85 square kilometres (33 sq mi) of floating ice in 2000–2001, followed by a 28-square-kilometre (11 sq mi) iceberg breaking off in 2008, and then a 260 square kilometres (100 sq mi) iceberg calving from ice shelf in August 2010. This became the largest Arctic iceberg since 1962, and amounted to a quarter of the shelf's size. In July 2012, Petermann glacier lost another major iceberg, measuring 120 square kilometres (46 sq mi), or twice the area of Manhattan. As of 2023, the glacier's ice shelf had lost around 40% of its pre-2010 state, and it is considered unlikely to recover from further ice loss.
In the early 2010s, some estimates suggested that tracking the largest glaciers would be sufficient to account for most of the ice loss. However, glacier dynamics can be hard to predict, as shown by the ice sheet's second largest glacier, Helheim Glacier. Its ice loss culminated in rapid retreat in 2005, associated with a marked increase in glacial earthquakes between 1993 and 2005. Since then, it has remained comparatively stable near its 2005 position, losing relatively little mass in comparison to Jakobshavn and Kangerlussuaq, although it may have eroded sufficiently to experience another rapid retreat in the near future. Meanwhile, smaller glaciers have been consistently losing mass at an accelerating rate, and later research has concluded that total glacier retreat is underestimated unless the smaller glaciers are accounted for. By 2023, the rate of ice loss across Greenland's coasts had doubled in the two decades since 2000, in large part due to the accelerated losses from smaller glaciers.
Processes accelerating glacier retreat

Since the early 2000s, glaciologists have concluded that glacier retreat in Greenland is accelerating too quickly to be explained by a linear increase in melting in response to greater surface temperatures alone, and that additional mechanisms must also be at work. Rapid calving events at the largest glaciers match what was first described as the "Jakobshavn effect" in 1986: thinning causes the glacier to be more buoyant, reducing friction that would otherwise impede its retreat, and resulting in a force imbalance at the calving front, with an increase in velocity spread across the mass of the glacier. The overall acceleration of Jakobshavn Isbrae and other glaciers from 1997 onwards had been attributed to the warming of North Atlantic waters which melt the glacier fronts from underneath. While this warming had been going on since the 1950s, 1997 also saw a shift in circulation which brought relatively warmer currents from the Irminger Sea into closer contact with the glaciers of West Greenland. By 2016, waters across much of West Greenland's coastline had warmed by 1.6 °C (2.9 °F) relative to 1990s, and some of the smaller glaciers were losing more ice to such melting than normal calving processes, leading to rapid retreat.
Conversely, Jakobshavn Isbrae is sensitive to changes in ocean temperature as it experiences elevated exposure through a deep subglacial trench. This sensitivity meant that an influx of cooler ocean water to its location was responsible for its slowdown after 2015, in large part because the sea ice and icebergs immediately off-shore were able to survive for longer, and thus helped to stabilize the glacier. Likewise, the rapid retreat and then slowdown of Helheim and Kangerdlugssuaq has also been connected to the respective warming and cooling of nearby currents. At Petermann Glacier, the rapid rate of retreat has been linked to the topography of its grounding line, which appears to shift back and forth by around a kilometer with the tide. It has been suggested that if similar processes can occur at the other glaciers, then their eventual rate of mass loss could be doubled.
There are several ways in which increased melting at the surface of the ice sheet can accelerate lateral retreat of outlet glaciers. Firstly, the increase in meltwater at the surface causes larger amounts to flow through the ice sheet down to bedrock via moulins. There, it lubricates the base of the glaciers and generates higher basal pressure, which collectively reduces friction and accelerates glacial motion, including the rate of ice calving. This mechanism was observed at Sermeq Kujalleq in 1998 and 1999, where flow increased by up to 20% for two to three months. However, some research suggests that this mechanism only applies to certain small glaciers, rather than to the largest outlet glaciers, and may have only a marginal impact on ice loss trends.

Secondly, once meltwater flows into the ocean, it can still impact the glaciers by interacting with ocean water and altering its local circulation - even in the absence of any ocean warming. In certain fjords, large meltwater flows from beneath the ice may mix with ocean water to create turbulent plumes that can be damaging to the calving front. While the models generally consider the impact from meltwater run-off as secondary to ocean warming, observations of 13 glaciers found that meltwater plumes play a greater role for glaciers with shallow grounding lines. Further, 2022 research suggests that the warming from plumes had a greater impact on underwater melting across northwest Greenland.
Finally, it has been shown that meltwater can also flow through cracks that are too small to be picked up by most research tools - only 2 cm (1 in) wide. Such cracks do not connect to bedrock through the entire ice sheet but may still reach several hundred meters down from the surface. Their presence is important, as it weakens the ice sheet, conducts more heat directly through the ice, and allows it to flow faster. This recent research is not currently captured in models. One of the scientists behind these findings, Alun Hubbard, described finding moulins where "current scientific understanding doesn’t accommodate" their presence, because it disregards how they may develop from hairline cracks in the absence of existing large crevasses that are normally thought to be necessary for their formation.